Topic 1.1: Introduction to Thermodynamics
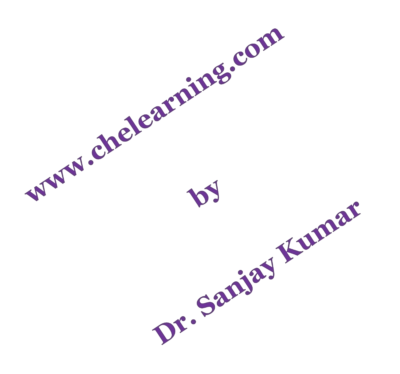
Historical Background
It was started a long time ago to describe the operation of a steam engine.
- Thermo – related to temperature or heat
- Dynamics – related to motion, force, or power
- It means how power can be developed out of heat. For example, fuel gets burned in vehicles and produces heat. This heat is used to power the vehicle.
Thermodynamics deals with work, heat, and energy and tells its effect on the system. It occurs in our day-to-day events. Governing physics for these processes is based on nature’s fundamental laws. Either we observe these laws or understand them via experiments.
Scopes and Limitations of Thermodynamics
All processes such as natural or other processes which we cause to occur are subjected to the law of thermodynamics.
- It tells that a process will occur or not under certain conditions.
- It tells/determines the state of equilibrium under given conditions.
- It tells the direction in which changes take place in nature.
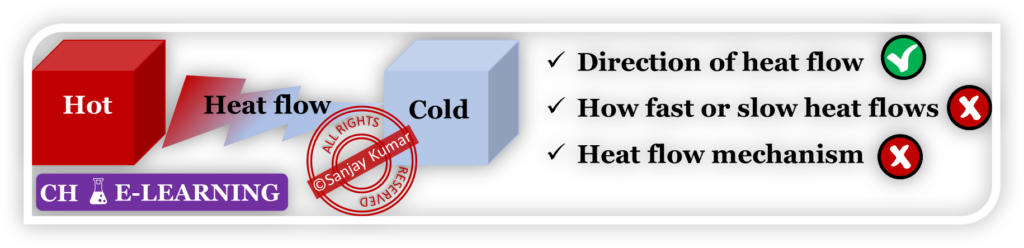

- It doesn’t tell the rate at which process occurs. Because the rate is a function of driving force and resistance. Thermodynamics variables are only responsible for diving force, not resistance.
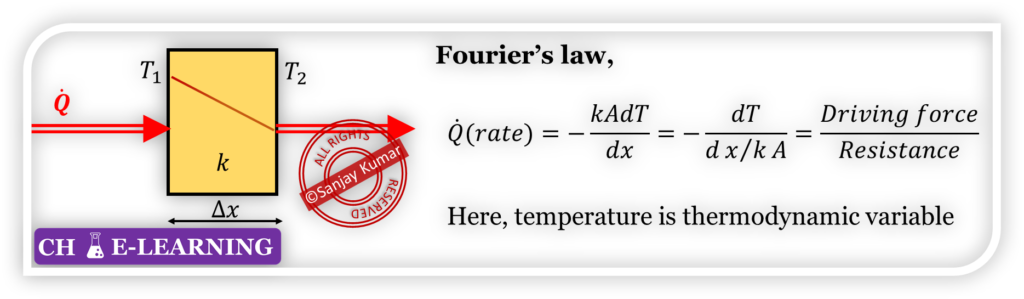
- It doesn’t tell the mechanism (which means heat flows via conduction/convection/radiation) of the process. Only deals with the initial and final state.
Importance in Chemical Engineering
Thermodynamics plays an important role in the chemical industry for understanding energy and matter interaction in chemical processes. It helps
- In the design of unit processes (chemical processes) and unit operations (separation units).
- In estimating heat and work requirements for physical and chemical processes.
- In estimating the minimum reflux ratio in the distillation column to achieve a desired separation.
- In determining conditions of equilibrium for both physical and chemical changes.
- In determining the feasibility of chemical reactions.
- In estimating the maximum yield obtained under given conditions of T & P.
- It tells that chemical reaction conversion above the equilibrium value could be achieved.
- In selecting optimum conditions for the reactor such as T, P, concentrations of reactant, etc.
Case Study
Production of iron (Fe) from iron ore (Fe2O3) in a blast furnace.
Process Description:
Iron is produced in a blast furnace by the reduction of iron ore (Fe2O3) using carbon monoxide (CO) as a reducing agent.
Raw Materials: In the blast furnace, iron ore, coke, and limestone are used as raw materials.

- Iron ore contains impurities such as sand (SiO2). To take care of impurities, limestone is used along with raw materials.
- The iron ore must be reduced using a stronger reducing agent (CO), which removes oxygen from the ore. For this purpose, coke is used along with raw materials.
Preheating & Charging: The raw materials are preheated to increase their temperature and reactivity.
- The preheated materials are charged into the top of the blast furnace, while hot air is introduced at the bottom.

Generation of Reducing Agent (CO): Coke reacts with hot oxygen to form carbon dioxide.
C+O_2\rightarrow CO_2- The CO2 formed then reacts with the remaining coke present in the furnace to generate CO, the reducing agent.
Reduction of Iron Ore: The generated CO reacts with iron ore (Fe2O3) to produce iron (Fe) and carbon dioxide.
Fe_2O_3+3CO\rightarrow2Fe(s)+3CO_2(g)Removal of Impurities (Slag Formation): Limestone (CaCO3) undergoes thermal decomposition to form calcium oxide and carbon dioxide.
CaCO_3\rightarrow CaO+CO_2- The CaO produced reacts with impurities such as SiO2 (sand) to form calcium silicate (slag).
- The slag, being less dense than molten iron, floats on top and can be removed.
Problem During Early Development:
In the early development of blast furnace technology, carbon monoxide (CO) was detected in the effluent gases.
- Initial Understanding: It was initially believed that the presence of CO was due to insufficient contact time between the iron ore and CO inside the furnace.
- Attempted Solution: Taller furnaces (30-40 meters) were built to increase the residence time of the reactants, believing that this would lead to improved iron conversion.
- Outcome: However, this approach did not significantly enhance the iron conversion process because the role of chemical equilibrium in chemical reactions was not fully understood at the time.
Relevance of Thermodynamics:
Later, the application of thermodynamic principles revealed that the reactions occurring within the furnace are governed by chemical equilibrium.
- Equilibrium Concept: Once equilibrium is reached, no further reaction takes place, it is impossible to increase the conversion of iron beyond this point, no matter how long the reactants are in contact.
- Temperature Influence: At high temperatures (above 1000 °C), CO predominates, enhancing the reduction of iron oxides, whereas at lower temperatures, CO2 formation is favored, reducing the effectiveness of the process.
- Technological Improvements: Understanding this led to significant improvements in furnace design and operation by focusing on optimizing temperature zones, gas flow, and material flow to achieve higher efficiency. This also helped in reducing CO emissions in effluent gases, rather than just focusing on increasing residence time.
Conclusion:
This case study emphasizes how the understanding of thermodynamics and chemical equilibrium is crucial in optimizing industrial processes, like the production of iron in a blast furnace.
Thermodynamic Approaches
Thermodynamics can be understood through two distinct approaches:
Classical Thermodynamics | Statistical Thermodynamics |
---|---|
It is based on a microscopic approach | It is based on a microscopic approach |
The behavior of individual particles is not required | The behavior of individual particles is required |
It depends upon the molecular structure of the matter | It depends upon the molecular structure of the matter |
Matter is continuous | Matter is particulate |